Caleb D. Alterman, Anton D. Perera, Prateek K. Gupta, and Daniel M. Alterman,
Germantown, Tennessee
Background: Endovascular treatment of peripheral arterial disease requires safe and reliable arterial access. This study evaluates the feasibility and safety of percutaneous axillary artery ac-
cess for endovascular therapy. A variety of anatomic and logistic obstacles can be overcome with upper extremity access. In this retrospective case review, we describe our experience
with percutaneous trans-axillary access for lower extremity peripheral arterial disease
intervention.
Methods: Medical records of all patients undergoing axillary artery percutaneous access from
December 2021 to August 2024 were reviewed. Demographic data, procedural details, and
complications such as pseudoaneurysm, hematoma, nerve injury, and closure device success
were analyzed. Technical success and perioperative complicationsdincluding bruising, edema,
hematoma, nerve injury, infection, thrombosis, and pseudoaneurysmdwere assessed. Clinical
outcomes were analyzed via follow-up clinic records.
Results: During the study period, 79 axillary artery accesses were performed on 64 patients.The patients were 55% male and had typical vascular comorbidities: hypertension (87%), hyper-
lipidemia (68%), coronary artery disease (27%), stroke (19%), and diabetes (16%); 58% were active tobacco users, and 80% were former tobacco users. Axillary access facilitated peripheral
endovascular procedures, including iliac intervention (55), femoral (44), mesenteric (16), tibial
(11), and embolization or visceral aneurysm treatments. A 6F sheath and ultrasound-guided
Angio-Seal closure device were uniformly employed, with no major complications, perioperative
deaths, or reoperations required. Minor complications of bruising and edema were present in 11 patients (14%). Other minor complications such as hematoma, nerve injury, thrombosis, dissec-
tion, pseudoaneurysm, or limb ischemia were absent.
Conclusions: Percutaneous axillary artery access demonstrates promise for complex endo-
vascular interventions with a favorable safety profile. Advantages include avoidance of unfavor-
able femoral anatomy, improved working length compared with radial access, and enhanced control of visceral therapy. Bilateral iliac and lower extremity therapy is feasible in a single treat-
ment as well. Percutaneous axillary artery access is a safe, reliable adjunct for enhancing endo-
vascular arterial intervention capabilities.
INTRODUCTION
Percutaneous arterial interventions have tradition- ally utilized the common femoral artery as the primary access site.1 However, alternative vascular access sites become essential when femoral anatomy is challenging or compromised. Alternative vascular access sites include brachial, radial, or tibial locations. Upper extremity access, including the axillary artery, is increasingly employed in cases of hostile femoral anatomy, infection, vessel angula- tion, and existing bypass grafts. This approach is particularly valuable in complex endovascular pro- cedures where bilateral femoral access proves inad- equate for multiple deployment systems.2 Despite its possible advantages, axillary artery access raises concerns about potential complications such as brachial plexus injury, pseudoaneurysm for mation, and thrombosis.3 Alternative approaches like the brachial and radial arteries are well docu mented, with the brachial artery associated with a complication rate of up to 11%. In contrast, the radial approach generally has a lower complication rate of about 2e3%.4 Radial artery access is used routinely for various arterial interventions but the lower extremity evaluation often necessitates longer working device or wire lengths and may impose challenges related to haptic control. Existing literature on trans-axillary access primarily focuses on open exposure for cardiac valve delivery and complex aortic aneurysm repairs. Studies have shown that up to 13% of patients undergoing aneurysm repair have iliac anatomy unsuitable for endovascular treatment.5 Additionally, upper extremity access facilitates procedures involving mesenteric or renal vessels.6,7 Recent descriptions of percutaneous axillary artery access for endovascular interventions reported a 1% mortality and 14% complication rate. The reported mortality was attributed to the complexity of the index procedure, which was complex endovascular aortic aneurysm repair.
This is a retrospective review of single-center experience with percutaneous axillary access for peripheral arterial intervention.
METHODS
We examined medical records of patients who underwent percutaneous axillary artery access for endovascular interventions between December 2021 and August 2024 at the Vascular and Vein Institute of the South. Procedures were performed in an outpatient treatment facility. We reviewed operative reports and clinical follow-up documentation to collect data on basic patient demographics, smoking status, medical comorbidities, medications, and outcomes. Axillary access was chosen as the primary alternative when femoral or pedal access sites were deemed unsuitable. To avoid the need for extended For infrainguinal evaluation, the radial artery was not used. Standard exclusion criteria for femoral access included dense femoral scar tissue, significant femoral artery calcification, extensive occlusive disease of the common femoral artery, morbid obesity with excessive vessel depth, and the presence of an aortobifemoral graft or endovascular aortic stent grafts that precluded crossover treatment. Axillary artery access was determined based on physician examination and ultrasound characteristics, with the left axillary artery being used exclusively. Selection criteria for axillary access included left axillary artery only, palpable upper extremity pulses, target vessel depth less than 3 cm as assessed by ultrasound, and no excessive calcification or occlusive disease in the axillary artery. All procedures were performed under moderate sedation. We employed ultrasound-guided percutaneous axillary artery access using a micropuncture needle, followed by upsizing to a 6F sheath over a Terumo Glide Wire (Terumo Interventional Systems) using the Seldinger technique (Figs. 1 and 2). To minimize the risk of pleural injury, the axillary artery was accessed between the lateral border of the first rib and the pectoralis minor muscle, visualized with ultrasound to avoid the axillary vein located anteriorly and superiorly. Arterial closure was performed using an Angio-Seal (Terumo Interventional Systems) vessel closure device deployment with ultrasound guidance in all cases. Ultrasound-guided access with a micropuncture needle was utilized, ensuring the puncture was limited to the 12 o’clock position with a single anterior entry. Needle and microwire positions were confirmed before advancing to a micro sheath and then upsizing to a 6F sheath. A retrograde axillary angiogram was conducted to verify the position and exclude aberrant anatomy or occlusive disease (Fig. 3). Systemic heparinization followed successful access and the decision to intervene. Fluoroscopic guidance was used to navigate to the descending thoracic aorta, avoiding manipulation of the aortic arch and proximal aorta. The closure technique involved longitudinal visualization of the Angio-Seal device with ultrasound-guided deployment, and deployment adequacy was confirmed with both B-mode and duplex ultrasound assessment (Fig. 4). Chronic anticoagulation was suspended 2 or 5 days preprocedure depending on the use of novel oral anticoagulants or warfarin therapy. For all procedures, systemic heparinization with 100 units/kg was used.
Heparin was administered after the decision to intervene, with partial redosing if the procedure time exceeded 1 hour. As 98% of therapy involved the treatment of arterial occlusive disease, dual antiplatelet therapy was advised for 30 days, with maintenance of aspirin therapy thereafter. For patients currently on clopidogrel, this was not suspended for the procedure and was continued. For those patients not previously on clopidogrel, just aspirin therapy, a loading dose of 300 mg was given postprocedurally after confirming access closure success and stability. An immediate duplex ultrasound assessment determines access closure success before transfer to the recovery area. Stability is determined by clinical assessment of the access site for a minimum observation time of 45 minutes and then according to anesthetic recovery needs. Postprocedure instructions include using a sling for 24 hours and keeping the ipsilateral arm to the side. The patients are instructed not to elevate the arm over the shoulder level or lift more than 5-10 lbs. on the same side. Periprocedural heparin is not reversed. In our experience, this is rarely needed and avoids the rare but severe protamine side effects and reactions. Procedures were categorized into 6 types: visceral aneurysm repair procedures, mesenteric vessel intervention, iliac intervention, femoral intervention, tibial intervention, and embolization therapy. Outcomes were assessed based on technical success, defined by successful cannulation of the target vessel and completion of the intended treatment. Significant complications were defined as perioperative events (occurring within 30 days) such as. Stroke, significant bleeding, need for operative repair of access site, or death were considered significant complications. Minor complications included hematoma, nerve injury, thrombosis, dissection, pseudoaneurysm, and limb ischemia. Postprocedural bruising or edema was also considered a minor complication. The standard follow-up included a postoperative clinic visit approximately 3 weeks after the procedure, followed by imaging at 1 month and 3 months.
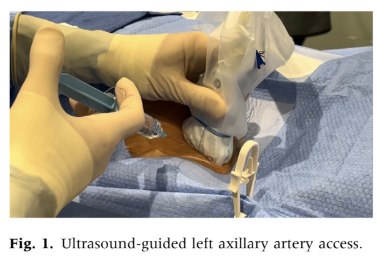
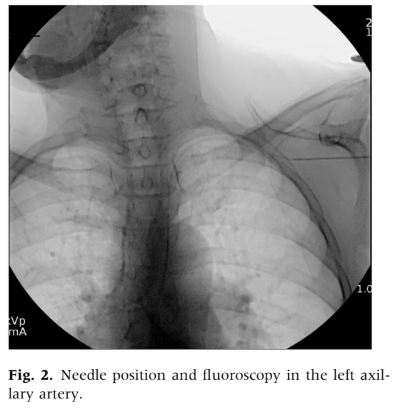
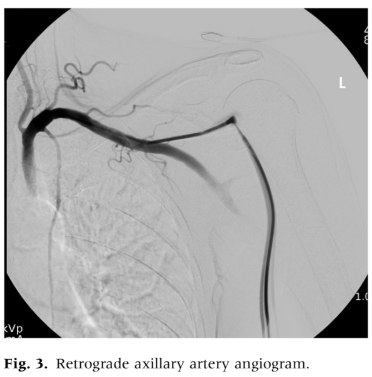
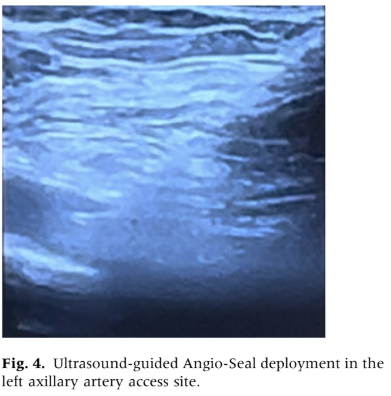
RESULTS
Over 33 months, we performed 1,400 peripheral arterial procedures. Out of these 1,400 arterial cases reviewed, 79 axillary artery punctures were performed in 64 patients for peripheral endovascular interventions. The cohort had a mean age of 70 years, with a mean height of 1.7 ± 0.09 m, weight of 69 ± 14.2 kg, body mass index of 24 ± 5.16 kg/m², and a baseline creatinine of 1 ± 0.02 mg/dl. The majority of patients were male (55%). The group exhibited a typical vascular comorbidity profile: hypertension (87%), hyperlipidemia (68%), coronary artery disease (27%), myocardial infarction (27%), stroke (19%), diabetes (16%), chronic obstructive pulmonary disease (14%), chronic kidney disease (10%), chronic heart failure (6%), and end-stage renal disease (1%). Tobacco use was prevalent, with 80% being former users and 58% current users. The vascular medication profile included aspirin (73%), statins (65%), clopidogrel (61%), and anticoagulants (13%). Additionally, 1% of patients were on dialysis with arteriovenous access on the right side, which did not interfere with axillary access. A total of 4% had a history of endovascular aneurysm repair, 3% had aortobifemoral bypass, and 1% had a left-sided cardiac pacemaker (Table I). We have avoided access ipsilateral to a dialysis access due to concerns related to interference with flow dynamics as well as preservation of the dialysis circuit. Our proposed anatomic criteria for percutaneous axillary artery access are given in Figure 5. In 73 punctures (92%), intervention was performed. On average, 2 vessels were treated per access. The most common intervention sites included: right common iliac artery (25 patients, 32%), right external iliac artery (24 patients, 30%), left common iliac artery (20 patients, 25%), left external iliac artery (18 patients, 23%), right femoral artery (15 patients, 19%), and left superficial femoral artery (10 patients, 13%). Ultrasound guidance was employed for both access and closure uniformly. The mean procedure time was 52 minutes.
Table I. Baseline characteristics
Baseline characteristics | N = 79 |
---|---|
Basic Demographics | |
Age, year, mean (±SD) | 70 (±6.5) |
Male | 55% |
Height, meter, mean (±SD) | 1.7 (±0.1) |
Weight, kilogram, mean (±SD) | 69 (±14) |
BMI, kilogram-square meter, mean (±SD) | 24 (±5.2) |
Baseline creatinine, mg/dl, mean (±SD) | 1 (±0.4) |
Comorbidities | |
HTN | 87% |
HLD | 68% |
CAD | 27% |
MI | 27% |
CVA | 19% |
DM | 16% |
COPD | 14% |
CKD | 10% |
CHF | 6% |
ESRD | 1% |
Tobacco usage | |
Current tobacco usage | 58% |
Former tobacco usage | 80% |
Medication | |
Aspirin therapy | 73% |
Statin therapy | 65% |
Clopidogrel therapy | 31% |
Anticoagulation therapy | 13% |
Surgical history | |
Hemodialysis fistula creation | 1% |
Fistula site not ipsilateral to axillary access | 100% |
History of EVAR | 4% |
History of Aortobifemoral bypass | 3% |
Presence of cardiac pacemaker | 1% |
Cardiac pacemaker left sided if present | 100% |
SD = standard deviation; BMI = body mass index; HTN = hypertension; HLD = hyperlipidemia; CAD = coronary artery disease; MI = myocardial infarction; DM = diabetes mellitus; CVA = cerebrovascular accident; COPD = chronic obstructive pulmonary disease; CKD = chronic kidney disease; CHF = chronic heart failure; ESRD = end-stage renal disease; AVF = arteriovenous fistula; AVG = arteriovenous graft; UE = upper extremity; EVAR = endovascular aneurysm repair.
Values are reported as % unless otherwise indicated.
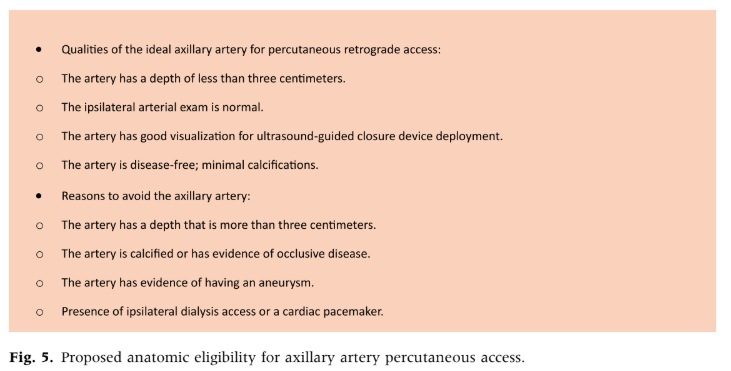
Fluoroscopy time was 11 minutes, and the radiation dose was 115 milli-gray (Table II). Technical success with closure was achieved in all cases. The average follow-up period was 19 days, with a total follow-up duration of 173 days. No major complications, including perioperative death or return to the operating room, were observed. Minor complications, such as hematoma, nerve injury, thrombosis, dissection, pseudoaneurysm, limb ischemia, and infection, were also absent, except for several instances of bruising and edema (Table III). Technical success for closure was uniform. Major complications are listed as absent and are also reiterated in Table III. Table III defines both major and minor complications, with a total of 6 instances of postprocedure ecchymosis at the access site and adjacent chest wall being observed on follow-up. Mild limb edema noted on follow-up was present in five patients. These were listed as minor complications in that they were all self-limiting and did not require any specific therapy.
DISCUSSION
Endovascular techniques for vascular disease are evolving, with upper extremity access, particularly through the axillary artery, becoming increasingly utilized. This approach offers distinct advantages, such as bypassing challenging femoral anatomy and negating the need for longer wires and devices. Our study found no significant neurological or ischemic complications associated with this method.
Compared to transradial or brachial artery access, axillary access provides several benefits: it allows for a greater working length of wires and devices, enhancing control over pushability, torque, and proximity to the target vessel. This is especially advantageous for infrainguinal treatments with prior placement of endovascular aortic aneurysm repair or aortobifemoral bypass grafts, which may be impractical with contralateral femoral access. Additionally, axillary access facilitates the treatment of acutely angled visceral vessels and enables simultaneous bilateral iliac and femoral interventions (Fig. 6).
We propose selection criteria for axillary artery access with ultrasound guidance for both initial access as well as closure device deployment. Anatomic considerations, experience, and lessons learned have guided this selection (Fig. 5). We believe closure device success is related to target vessel selection, with an ideal axillary artery being disease-free, with a depth of less than 3 cm and minimal tortuosity. If closure device deployment were not successful, simple maneuvers such as direct pressure and heparin reversal could be applied. While our recent technical success rate has been high, early in our experience, some closure failures were managed with direct pressure with success. Additional bailout maneuvers include immediate access via a transradial (more expeditious) or transfemoral approach with either balloon occlusion or covered stent placement. Our technical success has been predicated on careful case selection and attention to what we feel are anatomic criteria for safe axillary artery access. While there are closure devices for sheaths larger.
Table II. Operative details
Operative details | No. (%) |
---|---|
Procedure type | |
Diagnostic study only | 6 (8) |
Intervention performed | 73 (92) |
Intervention type | |
Occlusive iliac treatment | 55 (70) |
Occlusive femoral treatment | 44 (56) |
Occlusive mesenteric treatment | 16 (20) |
Embolization treatment | 1 (1) |
Visceral aneurysm treatment | 1 (1) |
Quantity of vessels intervened on, mean (±SD) | 2 (±1.1) |
Laterality of intervention | |
Right common iliac artery | 25 (32) |
Right external iliac artery | 24 (30) |
Left common iliac artery | 20 (25) |
Left external iliac artery | 18 (23) |
Right femoral artery | 15 (19) |
Superior mesenteric artery | 9 (11) |
Bilateral common iliac artery | 6 (8) |
Right superficial femoral artery | 8 (10) |
Left posterior tibial artery | 5 (6) |
Left femoral artery | 5 (6) |
Bilateral external iliac artery | 4 (5) |
Left anterior tibial artery | 2 (3) |
Right common femoral artery | 2 (3) |
Right anterior tibial artery | 2 (3) |
Bilateral femoral artery | 1 (1) |
Left common femoral artery | 1 (1) |
Left internal iliac artery | 1 (1) |
Right internal iliac artery | 1 (1) |
Right posterior tibial artery | 1 (1) |
No intervention performed | 4 (6) |
Size of sheath | |
6 French | 79 (100) |
Vascular closure device | |
Angio-Seal | 79 (100) |
Vascular access site | |
Left axillary artery | 79 (100) |
Procedure time, minutes, mean (±SD) | 52 (±19.6) |
Fluoroscopy time, minutes, mean (±SD) | 11 (±4.8) |
Radiation dose, milli-gray, mean (±SD) | 115 (±151.1) |
Ultrasound guidance for vascular access | 79 (100) |
Ultrasound guidance for vascular closure | 79 (100) |
SD, standard deviation. Values are reported as % unless otherwise indicated.
Table III. Outcomes
Outcomes | No. (%) |
---|---|
Closure technical success | 79 (100) |
Time to follow-up, days, mean (±SD) | 19 (±35.2) |
Length of follow-up, days, mean (±SD) | 173 (±226.2) |
Major complications | |
Perioperative death (within 30 days) | 0 (0) |
Reoperation | 0 (0) |
Minor complications | |
Hematoma | 0 (0) |
Bruising | 6 (8) |
Edema | 5 (6) |
Nerve injury | 0 (0) |
Thrombosis | 0 (0) |
Dissection | 0 (0) |
Pseudoaneurysm | 0 (0) |
Limb ischemia | 0 (0) |
SD, standard deviation. Values are reported as % unless otherwise indicated.
Than 6Fr, we avoid upsizing beyond this. Based on our early experience with this technique, we would not recommend routine percutaneous axillary access for outpatient peripheral arterial intervention with access sheaths larger than 6Fr.
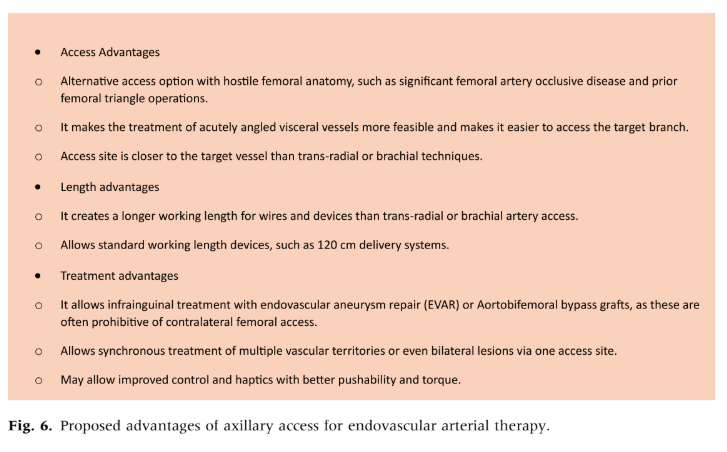
Previous literature for percutaneous axillary access is heterogeneous and comprises a variety of sheath sizes and treatment types including cardiac valve replacement, aortic aneurysm repair, and peripheral arterial disease therapy. We have refined our selection criteria and treatment algorithm over time to use this technique primarily for percutaneous treatment of peripheral arterial disease. Access to a shallow minimally diseased vessel combined with direct ultrasound-guided closure device deployment should achieve a high rate of technical success.
We insist on the access point being precisely anterior at the 12 o’ clock position. Sheath manipulation and passage of dilators should be done carefully, thus avoiding placing undue stress on the axillary artery access site. If the sheath were advanced over the wire with slight anterior torque, this could potentially dilate the access site and lead to a closure failure. This should be avoided. The access site should be carefully assessed for adjacent branches, such as the thoracoacromial artery; these may be inadvertently punctured during access or even interfere with closure device deployment. Taking care to clearly visualize these branches when selecting the access site may mitigate this risk.
Prior literature on percutaneous axillary artery access has predominantly focused on cardiac procedures and generally reports a low complication rate. Common complications noted include arterial dissection, bleeding or hematoma, and brachial plexus injury. Schäfer et al. reported an 11% complication rate in patients undergoing transcather aortic valve implantation via a trans-axillary approach, with bleeding being the most frequent issue. Modine et al. observed a similar pattern using the Proglide device, noting no strokes or major complications, but 7 out of 28 patients required a stent graft for minor bleeding, achieving a 100% closure success rate. This series is notable for using suture-assisted closure devices and 14 French delivery systems for deployment of transcatheter aortic valves.
In this retrospective case series, no major complications were observed. We found that ultrasound-guided Angio-Seal deployment for access closure in minimally diseased vessels achieved a high technical success rate. Precise access at the 12 o’clock position is a crucial technical step that contributes to successful closure. This underscores the importance of assessing the quality of the target vessel carefully and ensuring precise access.
While suture-mediated closure devices offer an option for hemostasis, our experience demonstrated that Angio-Seal consistently provides effective results. Our closure success rate with Angio-Seal was 100% across 79 punctures, which contrasts with the varied success rates reported in other studies. Although our complication rate was low, it is essential to recognize that all vascular closure devices carry some inherent risk of failure. Proper axillary artery puncture location is vital for facilitating exposure and clamp placement through a Standard infraclavicular incision. Having a reliable bailout strategy for potential access issues is also critical. The authors’ experience with this technique began in 2013. Our initial experience included using percutaneous axillary access with both a wide variety of clinical indications and 6, 7, and 8 Fr sheaths. While some percutaneous closure devices can accommodate larger sheaths, we found more reliable closure with 6 Fr access. We report our recent experience of building upon lessons learned and refining our selection criteria. Previously, we have used a variety of closure techniques, devices, and varying sheath sizes.
The distillation of our experience has culminated in the final iteration of our current technique involving primarily 6 French sheath access with ultrasound-guided Angio-Seal closure device deployment in those axillary arteries meeting our anatomic screening criteria. Our recent experience describes no major access complications with 100% technical success related to closure. There were 11 instances of both mild self-limiting bruising or site edema noted on early follow-up. These are described in Table III.
Inevitably, some access site failure will occur. We suggest techniques that we have previously employed with good success for management. Initially, direct pressure and reversal of anticoagulation when deemed appropriate are recommended. Despite not having an immediate posterior bone present to counterweight the compression, this is successful for small issues. Access site hematoma may arise when branches of the axillary artery are adjacent to the access site or even punctured during access, such as the thoracoacromial branch. For this reason, we try to carefully avoid adjacency of these branches and strive for anterior 12 o’clock locale for access.
Endovascular repair techniques that can be immediately applied include attempted delivery of a balloon and/or covered stent to the access site. This is often done most expeditiously via an ipsilateral transradial approach. Several commercially available covered stent grafts can be delivered via the radial artery. While more cumbersome, transfemoral access and delivery could be considered and has been used with success. Avoiding access selection ipsilateral to an existing dialysis circuit would thus avoid having to potentially instrument the dialysis access while attempting repair. This is part of the reason this is used as part of our anatomic access exclusion criteria (Fig. 5).
For delayed access complications such as pseudoaneurysm, ultrasound-guided thrombin injection could be considered provided it meets standard treatment criteria for this and is not associated with adjacent nerve or venous compression. Ultrasound-guided pseudoaneurysm thrombin injection can also be facilitated with a transradial delivered angioplasty balloon for temporary occlusion of its inflow during injection. Direct repair with axillary artery exposure may be rarely indicated if the above maneuvers are not successful. In our experience, this has been indicated twice in the prior 11 years; these cases are not given as they occurred early in our experience and not in the current description. One of these was for evacuation of a large hematoma occurring synchronous with a transcatheter-directed thrombolysis therapy. The other was related to a patient with a psychiatric history who pulled out his sheath while on a heparin infusion. Both cases were managed with direct exposure, hematoma evacuation, and repair.
Considering the rare but potential need for direct exposure, we avoid more medial axillary access, a diseased or calcified vessel, and an artery that is more than 3 cm deep with depth being preferably within 1-2 cm of the skin. If these suggested anatomic criteria are not observed, direct exposure would likely be much more challenging. Close postoperative monitoring of the access site and upper extremity is essential to detect potential complications, including axillary vein injury, thrombosis, brachial plexus injury (with or without hematoma), and axillary artery injury (Fig. 7).
CONCLUSION
Percutaneous axillary artery access for treating peripheral vascular disease has been demonstrated to be a viable and effective approach, with no significant complications reported, including stroke, brachial plexus injury, or ischemic events. This method is particularly beneficial in cases where femoral anatomy is challenging. Compared with radial artery access, there is less need for longer wires and devices, thus offering enhanced control and feasibility for infrainguinal treatments.
Compared to femoral, brachial, and radial access methods, axillary access provides a safe and practical alternative with notable logistic advantages. However, further research is essential to validate its safety profile and evaluate long-term outcomes.
CREDIT AUTHORSHIP CONTRIBUTION STATEMENT
Caleb D. Alterman: Writing – review & editing, Writing – original draft, Data curation.
Anton D. Perera: Writing – review & editing, Supervision, Conceptualization.
Prateek K. Gupta: Writing – review & editing, Validation, Supervision, Conceptualization.
Daniel M. Alterman: Writing – review & editing, Supervision, Methodology, Conceptualization.
REFERENCES
- Tayal R, Barvalia M, Rana Z, et al. Totally percutaneous insertion and removal of Impella device using axillary artery in the setting of advanced peripheral artery disease. J Invasive Cardiol 2016;28:374–80.
- Mallios A, Brown R, Blebea J. Axillary artery deployment of endovascular aortic repair iliac limb for preservation of internal iliac artery blood flow. Ann Vasc Surg 2016;31:246–51.
- Wooster M, Powell A, Back M, et al. Axillary artery access as an adjunct for complex endovascular aortic repair. Ann Vasc Surg 2015;29:1543–7.
- Harris E, Warner CJ, Hnath JC, et al. Percutaneous axillary artery access for endovascular interventions. J Vasc Surg 2018;68:555–9.
- Ahn SS, Feldtman RW, Hays FA. Thoracic aorta aneurysm repair using the right axillary approach. J Vasc Surg 2011;54:1201–4.
- Coscas R, de Blic R, Capdevila C, et al. Percutaneous radial access for peripheral transluminal angioplasty. J Vasc Surg 2015;61:463–8.
- Sos TA. Brachial and axillary arterial access, an overview of when and how these approaches are used. Endovascular Today 2010;55:55–8.
- Schäfer U, Ho Y, Frerker C, et al. Direct percutaneous access technique for transaxillary transcatheter aortic valve implantation: “the Hamburg Sankt Georg approach”. JACC Cardiovasc Interv 2012;5:477–86.
- Schäfer U, Deuschl F, Schofer N, et al. Safety and efficacy of the percutaneous transaxillary access for transcatheter aortic valve implantation using various transcatheter heart valves in 100 consecutive patients. Int J Cardiol 2017;232:247–54.
- Modine T, Obadia JF, Choukroun E, et al. Transcutaneous aortic valve implantation using the axillary/subclavian access: feasibility and early clinical outcomes. J Thorac Cardiovasc Surg 2011;141:487–491.e1.